Ammonia is one of the key raw materials in nitrogen-based fertilizers. This makes ammonia production co-responsible for growing 40% of the world’s food. With modern fertilizer manufacturing so heavily dependent on the Haber-Bosch process and its associated high energy consumption, global ammonia production accounts for more than 1% of energy-related carbon emissions.
If climate change is to be limited, a cheaper, greener way of producing ammonia is needed.
To this end, chemists have long been studying the enzyme nitrogenase which naturally produces ammonia at room temperature and ambient pressure. A key breakthrough in understanding how this catalyst works has now been made, one that may lead to the replacement of conventional fertilizer production, reducing environmental impact.
The discovery was made by a team based at a number of American universities but was centred on the Pacific Northwest National Laboratory in Washington State. Here, for the first time, the researchers were able to identify the elusive molecular structure inside nitrogenase that breaks nitrogen down to produce ammonia.
In technical circles, this structure is called the Janus intermediate; understanding and maybe even replicating this structure has long been held as a holy grail in the fertilizer industry.
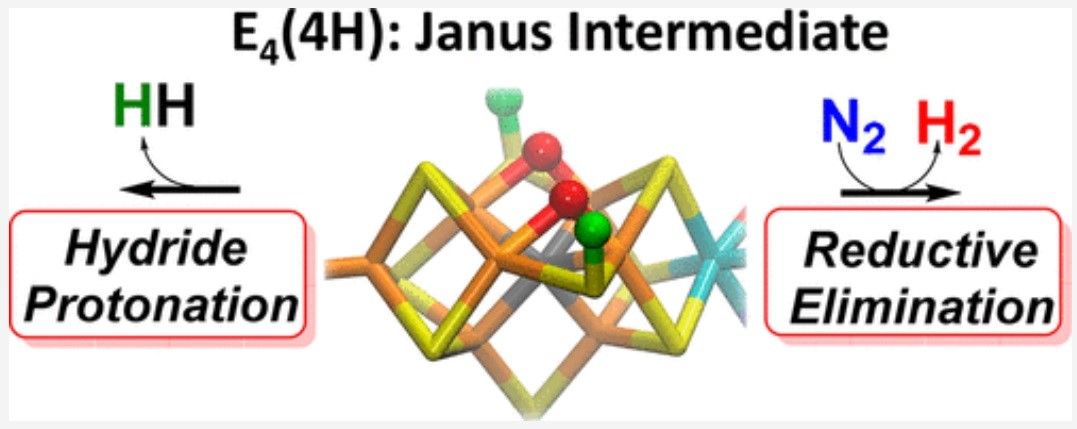
The challenge has always focused on how nitrogenase is able to break nitrogen’s bonds. As the scientific journal Phys.org reports, “For every molecule of nitrogen transformed to ammonia, nitrogenase makes at least one molecule of hydrogen (H2).” Something which Simone Raugei, a theoretical chemist and one of the study’s corresponding authors, describes as, “… one of the most puzzling mysteries of nitrogenase. Instead of just producing ammonia, you also produce this byproduct. Why is that necessary?”
Raugei and his colleagues found that this phenomenon was helping nitrogenase to break nitrogen's bonds.
“Nature found a solution by coupling the production of hydrogen, which releases energy, with cleaving nitrogen, which requires energy,” says Raugei. “It's total balance.”
As the PNNL press release explains, “… the structure of the Janus intermediate - particularly the spatial relationships of its electrons and protons - is important because it sheds light on how nitrogenase can store four electrons in a very small cluster of atoms to make breaking the strong chemical bond of nitrogen gas possible. Electrons naturally want to repel each other, so rotating them around in a confined space is tricky.”
“Understanding how to park four additional electrons in an already very electron-rich region is a true challenge for synthetic chemists,” states Raugei.
To do it, the team, “… used computer simulations coupled with a magnetic resonance analytic technique to explain the molecular and electronic structure of the unpaired electrons.”
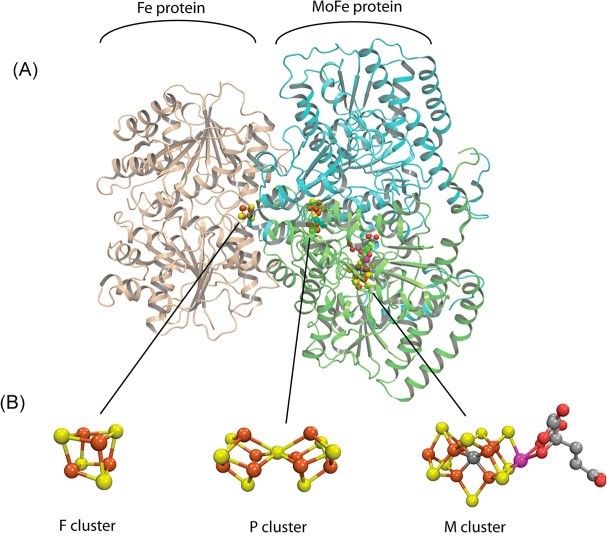
From this they were able to create a model of how the Janus intermediate functions, including the complex relationship between the nuclei and the electron cloud.
The team have now published details of their breakthrough in the Journal of the American Chemical Society where they report to, “… have shown that the key state in N2 reduction to two NH3 molecules by the enzyme nitrogenase is E4(4H), the ‘Janus’ intermediate, which has accumulated four [e–/H+] and is poised to undergo reductive elimination of H2 coupled to N2 binding and activation.”
“We were very well positioned to make to this breakthrough because we combined experimental information on nitrogenase with the computational information,” says Raugei. “That was key.”
Now that an improved understanding of the core principles employed by enzymes to directly control protons, electrons, and substrates has been made, work can proceed on creating a synthetic catalyst that can achieve the same specificities, efficiencies, and catalytic rates as nitrogenase.
Designing next-generation synthetic catalysts is at the heart of the modern chemical industry. If mankind is to limit its impact on climate change as well as increase food production over the coming years then it needs to find an economic and environmentally friendly way to produce ammonia. With this discovery, it is hoped that the biggest step to achieving this has been made.
Photo credit: PNNL, ACS, Journal of the American Chemical Society, KBR, & Soilsmatter