Like many addicts, acknowledging the addiction is the first and hardest step.
For this reason, it has taken the chemical industry many years to begin to see that its continued use of oil, gas, and coal is no longer sustainable and will soon no longer be acceptable. The world will still require all of the plastics, cosmetics, pharmaceuticals, paints, fertilisers, and other products that chemical companies manufacture, but will need it without the carbon emissions.
But what progress is being made and how is the chemical industry moving towards a more sustainable path?
Biopolymers
One of the biggest arguments against biopolymers is that the straightforward process of converting sugar or starch from a product like maize into a plastic uses up land that would otherwise grow food.
“This is something that is, unfortunately, sticking around, because it’s not true,” says Hasso von Pogrell, managing director of trade association European Bioplastics. “The amount is very low. Even if you anticipate high growth of bio-based plastics it is still very little.”
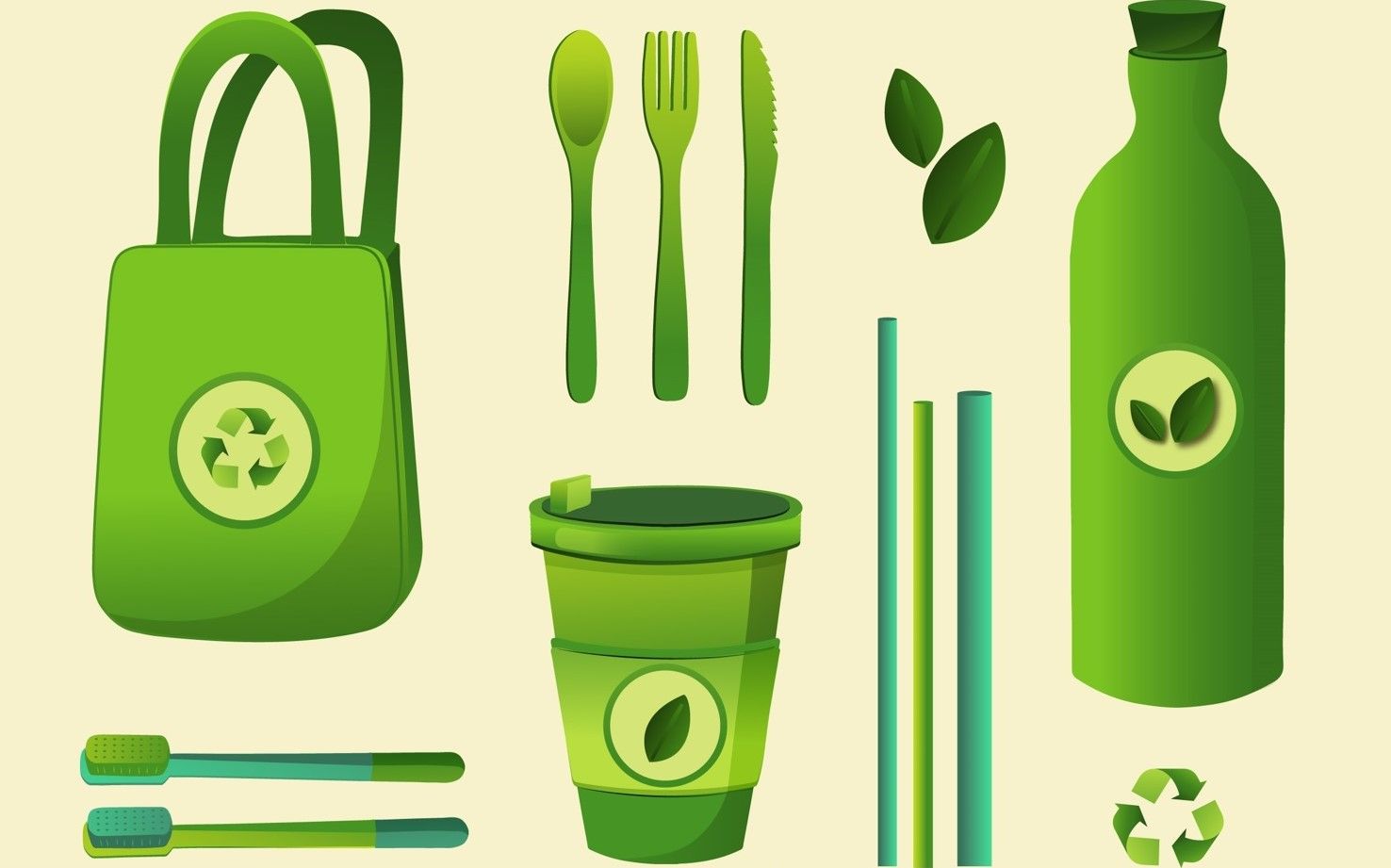
Instead, substantial amounts of biopolymers are sourced from waste products, such as trees thinned from forests, or unwanted timber mill sawdust and chippings.
The process is relatively simple and, more importantly, carbon neutral.
“The wood is first de-barked and chipped, and the chips treated with steam at high pressure and temperature, leading to a very fine pulp,” explains a report in the journal Chemistry World. “Cellulosic materials are extracted, mostly based on hemicellulose, or xylose if the source was a hardwood, and the pulp subjected to enzymatic hydrolysis. The lignin component of the wood remains a solid material. A catalytic hydrogenation process then cracks the sugar to give monoethylene glycol as the main product, and this is purified by distillation. It can then be used to make plastics such as polyethylene terephthalate (PET) for packaging and polyester textiles.”
Significantly, the lignin is not wasted; instead, it is transformed into a biodegradable functional filler that can replace silica and carbon black in rubber applications. Additionally, it can be used to create black plastic that can be recycled by optical sorting.
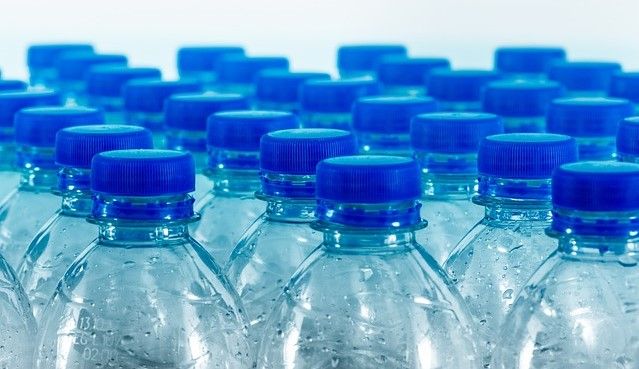
Alternatively, waste and residual oils and fats, such as used cooking oil, are converted into bio-based feedstock and fed into an existing steam cracker instead of traditional feedstocks like naphtha. In this process, the glycerol component of the oil is converted to propane during the hydrogenation process, and the fatty acids are transformed into linear alkane chains with 16–18 carbons for the cracker.
One of the biggest biochemical producer is Neste RE which has facilities making renewable diesel, sustainable aviation fuel, polymers, and chemicals in the Netherlands, Finland, and Singapore, as well as a joint venture in the US.
By early 2024, the company hopes to have an overall annual capacity of 5.5 million tonnes of bio-based chemical products. Such a large output is necessary, as potential customers worry about having enough available product from smaller or pilot plants.
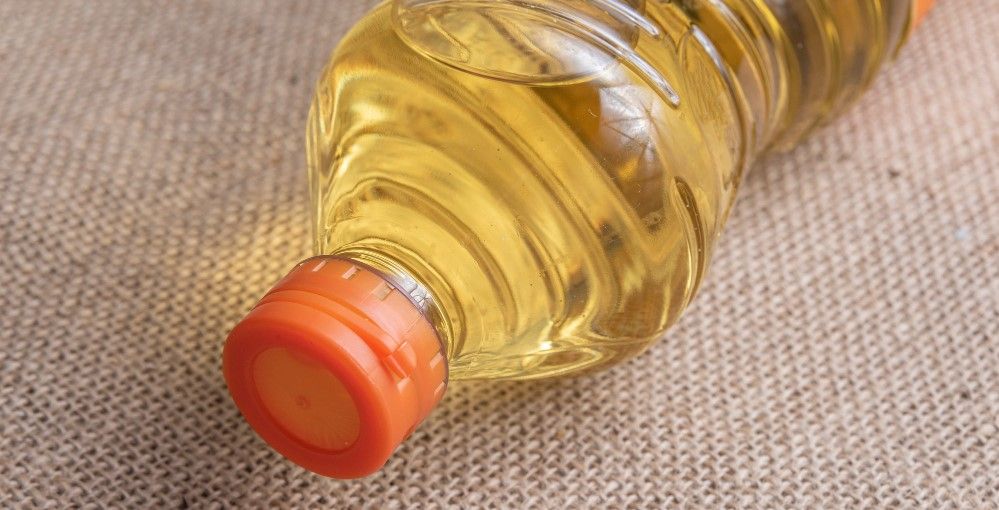
Close collaboration with customers has always been key for the company’s success.
“We discuss with brand owners what their needs are,” explains Lars Börger, a vice president for Neste renewable polymers and chemicals. “Then we connect them with production partners such as steam crackers to kick off more sustainable value chains that help the brand owners achieve their targets.”
One of the biggest challenges for many producers of biopolymers is that they are not ‘drop in’ replacements.
For example, polylactic acid (PLA), a polyester derived from starch-based crops such as corn, has had some success since industrial production began about thirty years ago. But their adoption requires some modification to the downstream process before they are useful. The same can be said of the bio-polyester polyethylene furanoate (PEF), but at least this product offers superior mechanical and barrier properties than the closely related PET. It can also be processed at lower temperatures.
Additional problems exist as both PLA and PEF both lack a current recycling stream. This can often discourage their uptake, as there is no way to reuse them. In a catch 22 situation, only by increasing their use will recycling become economically practical.
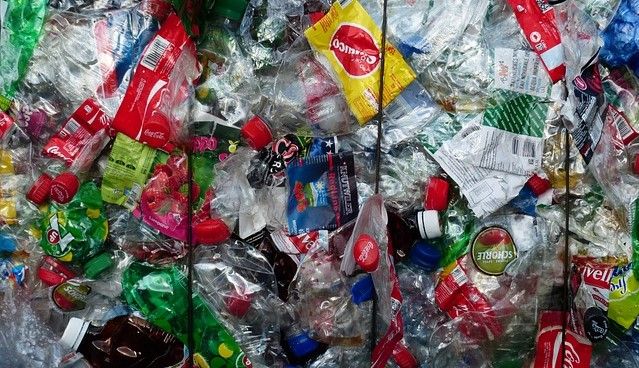
This has bioplastics producers calling for renewable materials to count towards recycled content targets in legislation.
“If you put the hurdle up too high or at the very beginning, it could be a killer for all the new bio-based materials, because how are you going to be recycling at scale if you’re not going to be on the market at scale?” notes von Pogrell. “We need to say it has to be recyclable, and you need to have time for it to scale up. Then you can say after 10 years, or even longer, it also has to show that it is recycled at scale.”
Chemical Recycling
There are already approximately 140 chemical recycling initiatives underway globally, although the majority of these are operating as pilot plants.
That said, some chemical companies are progressing well. For instance, the Carboliq company has a facility in Westphalia, which uses waste from a nearby recycling plant as input to create an industrially useful pyrolysis oil. Other facilities in Europe are already processing as much as 150,000 tonnes of material each year, with further plans for large plants underway at Lyondell Basell in Belgium and Dow Chemical and its partner Mura in Saxony.
However, compliance with EU regulation is often a hold up for these developments, with most agreeing that reform is needed for chemical recycling to move forward.
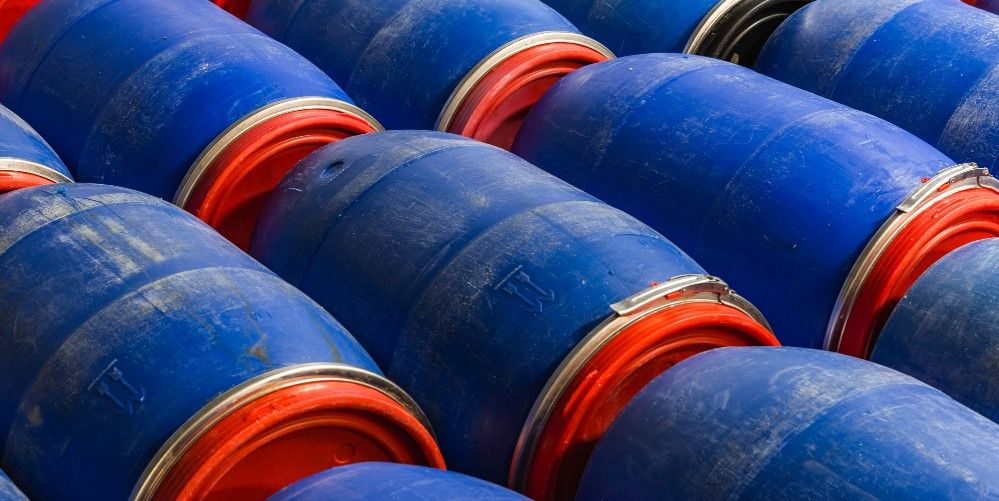
“The response of politicians, both in Europe and in Germany, is often that chemical recycling does not work at all,” explains Ingemar Bühler, Managing Director Plastics Europe. “That is simply nonsense. But there is also a fair criticism, namely that despite great progress, the energy input for chemical recycling is much higher than that for mechanical recycling. Mechanical recycling is highly efficient: PET bottles, for example, can be mechanically recycled several dozen times until the polymer structures can no longer withstand further use.”
But once the polymer chain has degraded so far, further mechanical recycling is no longer practical. Then the plastic is burnt, and the circularity ends. It is at this point in the process that chemical recycling can complete the circle and ensure continued use of the original raw material by converting it back to basic monomers.
For this reason, it is important to note that chemical recycling is not in competition with mechanical recycling. Instead, it is a way to avoid incineration.
“In today's legislation, the idea is to burn these polymers that are past their usability stage, and to generate energy from them,” says Bühler. “However, the cost of incineration is high, and the process releases CO2. Instead of incineration, it would be much better in our view to chemically recycle these polymers. In the best case, no CO2 is released, and the carbon continues to circulate.”
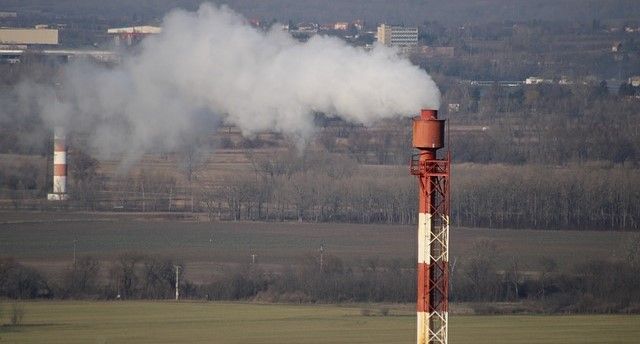
Conventional, fossil fuel-based polymers have been the raw material which built the modern age, but with 350 million tonnes of plastics produced every year, the chemical industry must either boost recycling or use more bio-based feedstocks for the entire process to remain sustainable.
As Michael Duetsch, vice president of UPM Biochemicals concludes, “Replacing petrochemicals with sustainable chemistry is a must-do for the next decade, but we face a gigantic challenge.”
Photo credit: Freepik, Freepik, Dávid Lehoczki from Pixabay, Wirestock, Hans, Willfried Wende, & User3802032